
Curator Chad Broyles (IODP-USIO/TAMU; back to camera), Expedition Project Manager and Staff Scientist Jörg Geldmacher (IODP-USIO/TAMU), Co-Chief Scientist Takashi Sano (National Museum of Nature and Science, Japan), and Co-Chief Scientist Will Sager (TAMU) discuss a core recovered from Shatsky Rise. (Credit: John Beck, IODP/TAMU)
Will Sager is a professor at Texas Agricultural and Mechanical University (TAMU) in College Station. When I invited him to speak he was bobbing about in a small boat in the Indian Ocean doing seismic surveys - and didn't seem quite as enthused about the experience as he expresses on his web page.
'Dr. Sager’s research is broadly about Earth history, plate tectonics, and the evolution of ocean basins. He is a marine geophysicist who loves to explore the ocean and has been on 38 research cruises and was chief scientist on 17 of them. He has participated on seven Ocean Drilling Program cruises and was co-chief scientist for two. His expeditions have taken him and his students to each of the big three oceans.'
Sager will be discussing the results from a recent cruise to the Shatsky Rise in the northwest Pacific where they were examining hot spot volcanism. Hot spots are taught in entry level plate tectonics classes as an important piece of evidence to support the global theory of plate tectonics. In geology, a hot spot is a portion of the Earth's surface that is far from tectonic plate boundaries where volcanism occurs due to a rising mantle plume or some other cause.

Figure showing the three basic types of volcanism on the planet and the mantle behaviour beneath those volcanoes.
Plumes were once thought to have formed most seamount chains, but today it is thought that classic, deep-rooted mantle plumes are rare. The 145 million-year-old Shatsky Rise seamount chain has some characteristics that fit the plume-head hypothesis however other scientists suggest its formation is linked to spreading ridges (B in the figure above - creation of new ocean floor) and a triple junction. Located ~1500 kilometers (930 miles) east of Japan, Shatsky Rise measures roughly the size of California. This underwater mountain chain is one of the largest supervolcanoes in the world: the top of Shatsky Rise lies three and a half kilometers below the sea surface, while its base plunges to nearly six kilometers below the surface. Shatsky Rise is composed of layers of basalt, with individual lava flows (now hardened) that are up to 23 meters thick.
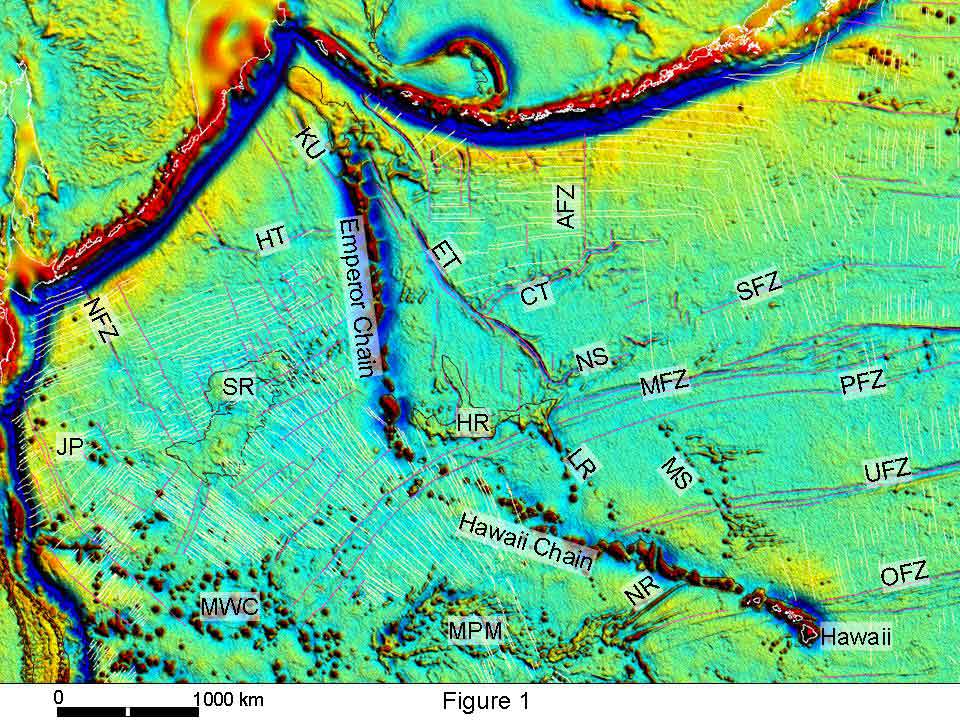
Map of the North Pacific showing the hotpot track of the Hawaiian and Emperor sea mount chains. To the west of the Emperor Chain is the Shatsky Rise (SR on the map). The map also shows trenches to the north and west of the chains.
According to Sager, Shatsky Rise is unique in that
'it is the only supervolcano to have formed during a time when Earth’s magnetic field reversed frequently.” This process creates “magnetic stripe” patterns in the seafloor. We can use these magnetic stripes to decipher the timing of the eruption and the spatial relationship of Shatsky Rise to the surrounding tectonic plates and triple junctions.'
On Friday Sager will discuss the expedition results, seamounts and plateaus of the Pacific, and his changing views on hotspots.