
This weeks speaker is Eric Steig from the University of Washington. Steig will be discussing climate change in Antarctica from the perspective of the ice sheets. Last year Steig was an author on a controversial paper published in Nature where they presented evidence that Antarctica is certainly warming. Scientists have known for a long time that the Antarctic Peninsula was warming rapidly causing ice shelves to break up (like the Larsen Ice Shelf) and glaciers to accelerate sliding toward the ocean. However, the East Antarctic Ice Sheet was previously believed to be cooling, creating a climate enigma because this finding runs counter to the forecasts of computer climate models. Part of the explanation for this result is because the gathering of weather data has occurred for a few decades in the sparsely populated East Antarctic. Over the last 20 to 30 years cooling has occurred over the East Antarctic, however, the reason for this cooling put forward by David Thompson (Colorado State) and Susan Solomon (NOAA Aeronomy Lab - the scientist who brought the attention of the Ozone Hole to the world) is the increasing strength of the circumpolar westerlies, and that this can be traced to changes in the stratosphere, mostly due to photochemical ozone losses. Substantial ozone loss did not occur until the late 1970s, and after this period significant cooling begins in East Antarctica.
In Steig's new study, satellite measurements were used to interpolate temperatures in the vast areas between the sparse weather stations, and the time interval studied was extended to 50 years. From 1957 through 2006, temperatures across Antarctica rose an average of 0.18 degrees Fahrenheit per decade, comparable to the warming that has been measured globally.
Steig says “We now see warming is taking place on all seven of the earth’s continents in accord with what models predict as a response to greenhouse gases”
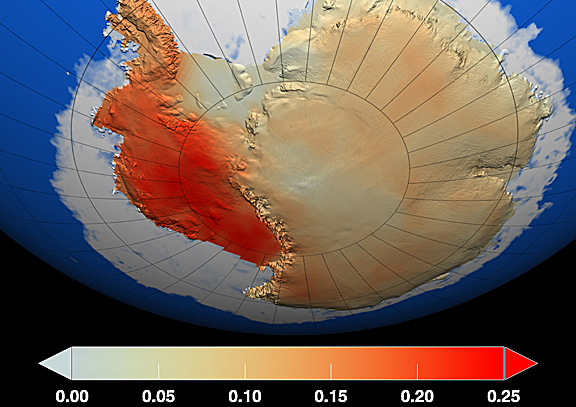
This caused ripples throughout the climate denier community. Global warming skeptics have pointed to Antarctica in questioning the reliability of the models because of the mismatch between the data and the models. A quick web search reveals accusations of fraud and bitter name calling. A blog that is supported by many top climate scientists to try and explain and debunk mnay of the issues raised by climate deniers is Real Climate. Have a look at the debate to get a sense of the passion in this field....Real climate debate
Below is the video produced by Nature on Steigs paper which is in your resources.